Faculty Profile
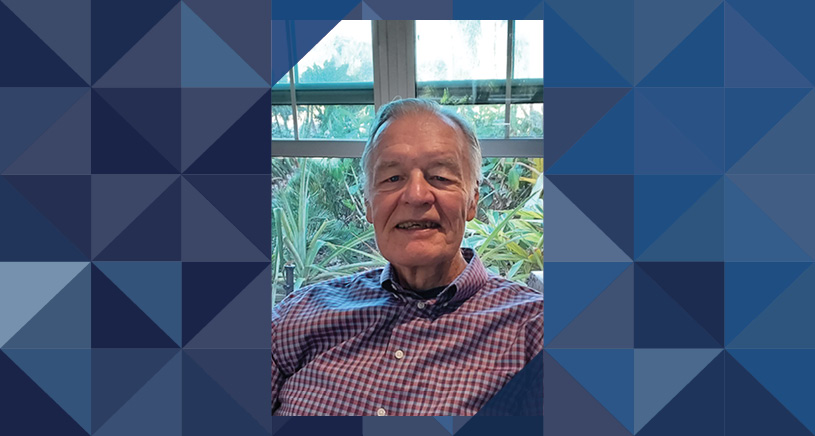
James S. Koopman, MD, MPH
- Professor Emeritus, Epidemiology
Jim has been oriented to advancing public health since he entered the University of Michigan medical school in 1963. He benefited from a joint studies program that gave him six years to complete medical school while simultaneously pursuing public health, social, political, and historical issues. He deepened his interest in Latin America during six months of medical training in Chile. After completing a pediatrics residency at Harbor General Hospital in 1972 he spent two years as an EIS officer and then acting state epidemiologist in the state of Washington. During the end of his EIS stint, he worked for WHO in smallpox eradication in India. He saw the last cases of smallpox ever in Azamgarh district of Uttar Pradesh and also saw the devastating effects of the vaccine that would never be tolerated today.
He then worked in Colombia, South America where he pursued new surveillance approaches
and training programs in the Cali health department with the support of CIDEIM. In
Cali he developed theories about infection transmission systems and
studied the philosophy and methods of mathematically modeling infection transmission.
He returned to Michigan as assistant professor of epidemiology in 1978, largely pursuing his practical field approach to epidemiology both in the state of Michigan and South America. After getting tenure, he took leave from the University for two years to work with CDC in initiating a national field epidemiology training and investigation service in Mexico that subsequently has had great success.
Upon returning to Michigan in 1986, Jim changed careers from field epidemiologist
to modeler and theorist. His colleagues John Jacquez and Carl Simon taught him some
of the basics. Initially he worked on HIV transmission systems and with his colleagues
demonstrated the potential of the early stages of HIV infection to accelerate the
population dissemination of infection. Then he switched to a series of other infectious
agents. His major focus, however, is not on agents, but on new methods for a science
of infection transmission system analysis. He is advancing two cornerstones of that
science. The first is the integration of diverse modeling methods into a single coherent
analytic approach called Inference Robustness and Identifiability Analysis (IRIA).
The second involves using microbiology laboratory studies and nucleotide sequences
of infectious agents to make inferences about transmission systems. He collaborated
with Carl Simon who was the first director of the Michigan Center for the Study of
Complex Systems and continues to view that center and Carl Simon as a focus of his
ongoing scientific work during his retirement.
- MD, University of Michigan, 1968
- MPH, University of Washington, 1975
Research Interests:
Jim's research focus is on developing theory and methods that promote a science of
infection transmission system analysis. He seeks to integrate microbiology, epidemiology,
social structure analysis, and complex systems science. Now in retirement, he continues
this quest by developing models of SARS-CoV-2 evolution and control through vaccination,
treatment, and public health actions. He seeks collaborators who want to pursue his
moto since 1986 of "Developing Theory that Serves the Public Health".
Research Projects:
His current project with Carl Simon formulates the contributions of diverse factors
to the evolution of SARS-CoV-2. A homogeneously mixing population ODE mathematical
structure is used to formulate the effects of mutations on drifting and waning of
immunity, transmissibility of the virus, escape from existing immunity, joint effects
of all pairs of mutations on transmissibility and immunity escape, and antibody dependent
enhancement (ADE) of transmission. The independent and joint effects of all these
factors on either transmission or evolution dynamics are difficult to intuit. The
current project is designed to advance such intuitions. It seeks abstract understanding
using simple formulations with the minimum number of mutation sites and alleles. That
should help improve the intuitions of modelers as they seek approaches to modeling
more complete biological and population structures affecting SARS-CoV-2 transmission
and evolution. It should also provide a first step toward using the massive amounts
of real world data that have been generated during the pandemic to estimate key parameter
values. That is where Koopman's work on IRIA will come into play.
Brouwer A.F., ..., Koopman J.S., ...(2022) The role of time-varying viral shedding in modelling environmental surveillance for public health: Journal of the Royal Society, Interface
Koopman, J.S. Simon, C., Getz, W.M., Salter, R. (2021). Epidemics Modeling the population effects of epitope specific escape mutations in
SARS-CoV-2 to guide vaccination strategies.
Koopman J.S., Henry CJ, Park JH, Eisenberg MC, Ionides EL Eisenberg JN (2017). Dynamics affecting
the risk of silent circulation when oral polio vaccination is stopped. Epidemics.
Koopman, J.S. (2017). Models and analyses to understand threats to polio eradication. BMC Medicine 15,21
Romero-Severson EO, Alam SJ, Volz E, Koopman J. (2013) Acute-stage transmission of HIV: Effect of volatile contact rates Epidemiology. 24: 516-521.
Koopman, J.S. (2007). Infection transmission through networks. In Kepes, F. (Ed.) Chapter 13 of Biological Networks. 1-58. World Scientific, Singapore
Koopman, J.S., Simon, C.P., Riolo, C.P. (September, 2005). When to control endemic infections by focusing on high-risk groups. Won the Rothman prize as the best paper published in Epidemiology in 2005. Epidemiology
621-627. Lippincott Williams & Wilkins.
Koopman, J.S. (2004). Modeling infection transmission. Annual Review of Public Health 303-326.
Koopman, J.S., Chick, S.E., Riolo, C.P., Simon, C.P., Jacquez, G. (2002). Stochastic effects of disseminating versus local infection transmission. Mathematical Biosciences 49-71.
Koopman, J.S., Simon, C.P., Jacquez, J.A. (1994). Assessing effects of vaccines on contagiousness and risk factors for transmission In EH and Brandeau ML (Eds.) (Ed.) Modeling the AIDS Epidemic: Planning, Policy,
and Prediction 439-460. Raven Press, New York
Koopman JS, Campbell J. (1975) The role of cutaneous diphtheria infections in a diphtheria epidemic Journal of Infectious Diseases. 131: 239-244.
Areas of Expertise: COVID-19, Infectious Disease, Public Health Practice